By Dave Linville, Vice President and General Manager, AquaSmart, Inc.
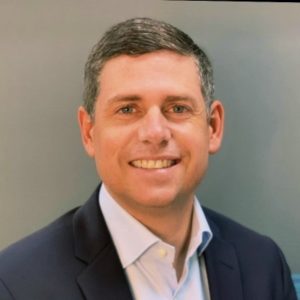
Throughout human history, lead pipes have been used to carry drinking water. In the US, it wasn’t until the 1980s that this practice was reconsidered for its potential harmful effects on humans. The original Lead and Copper Rule (part of the US Safe Drinking Water Act) was implemented in 1993, creating maximum concentrations of lead in drinking water.
In the late 1990s, in addition to setting maximum lead concentrations, the United Kingdom mandated the use of phosphoric acid to control lead corrosion in drinking water supply pipes. A minimum dose of 2.0 mg/l of phosphorus was required until utilities could prove lower concentrations were effective. Most utilities currently dose between 1.5 and 2.0 mg/l as phosphorus as a result of corrosion optimization studies.
Last decade, tens of thousands of residents of Flint, Michigan, were exposed to dangerous levels of lead (and other chemicals), which elevated public concern about lead poisoning from water. In response, the US EPA began the process of revising the Lead and Copper Rule, which goes into effect in 2024. An expected outcome of the 2024 rule revisions is an increased use of phosphoric acid for lead corrosion control in drinking water in the US. Wastewater treatment facilities, the phosphorus supply chain, and many aquatic ecosystems in the US are likely to be impacted as a result. After all, drinking water often becomes wastewater, carrying the phosphorus downstream.
In 2014, a study was conducted[1] to predict the impact on the Chesapeake Bay watershed if higher levels of phosphorus (such as currently used in the UK) were used for drinking water corrosion control in the US, and it found:
“The most common change to advanced treatment resulting from increased phosphorus loading was an increased addition of aluminum sulfate (88%), and the two most common changes to total solids disposal were an increase in the amount of total solids being disposed (83%) and an increase in the phosphorus concentration of the total solids being disposed (33%). The average annual cost increase resulting from phosphorus loading was $22,867/million gallons a day (MGD) for changes to advanced treatment and $17,164/MGD for changes to total solids disposal. While results showed that WWTPs can treat a phosphorus increase to 2 mg/L as P without violating TMDL permit levels, there will be a cost that every WWTP must determine and find a way to fund.”

As part of the US EPA 2016 OCCT Recommendation for Primacy Agencies[2], a study was cited[3] that concluded that as much as 35% of the phosphorus load in wastewater plants comes from use of corrosion control additives in drinking water. In the UK, where runoff is tightly controlled and use of phosphorus for corrosion control is higher, the amount of wastewater demand due to drinking water corrosion control is likely much higher than 35%.
In 2020 the phosphorus discharge limits in the UK were reduced, in some cases to as low as 0.1 mg/l, which created major challenges for wastewater operators. The number of sites in the UK requiring phosphorus treatment increased by 70%. Most of these sites are small and in densely populated areas, requiring complex logistics to build additional operational phosphorus removal capacity. The use of coagulants increased by >75%, requiring additional UK manufacturing capacity to produce them. Due to greater use of coagulants, larger volumes of pH adjustment chemicals became required, which contribute significantly to carbon footprint of a plant. In one study performed[4], the total costs of these changes were concluded to be “disproportionately expensive.” As a result of added cost and complexity, and an inability to reliably procure raw materials, implementation of the reduced discharge limits was delayed.
Responsible phosphorus use and reduced carbon footprints remain priorities for the entire industry. Every UK water utility has pledged to become carbon neutral by 2030. In response, utilities are beginning to deploy alternative technologies for corrosion control and coagulation. The use of poly-aluminum chloride to replace alum and ferric is gaining widespread adoption. The use of a water treatment additive, called SeaQuest, to replace phosphoric acid for corrosion control is being piloted due to its potential to reduce phosphorus use and carbon footprint significantly. The table below shows estimates from a UK water producer for the cost, phosphorus needs and carbon footprint of processes using phosphoric acid versus SeaQuest versus SeaQuest with no added lime (for pH control):

In the US, SeaQuest has been used successfully for corrosion control since the late 1990s. A study was performed to determine effectiveness at controlling lead release, and the results are included below. The 90th percentile lead results, in ug/l, as reported by drinking water utilities from 2002 to 2021, were obtained from a random selection of 63 SeaQuest users across 16 states, ranging in size from 0.1 MGD to 140 MGD of water production, including surface water, ground water and blended source water. SeaQuest utilizes a unique corrosion control mechanism and, as a result, requires significantly less added phosphorus to achieve corrosion control.


Atlanta-based AquaSmart, Inc. produces SeaQuest from 100% food-grade ingredients that continuously keep ortho-phosphate and polyphosphate in equilibrium, but without forming a layer of mineral scale inside of water pipes.
Opinions expressed here do not necessarily reflect those of the Sustainable Phosphorus Alliance.
[1] Cope, C. 2014. Implications of Phosphorus Treatment of Drinking Water for Significant Wastewater Treatment Plants in the Chesapeake Bay Watershed Portion of Virginia
[2] OCCT Evaluation Technical Recommendations for Primacy Agencies and Public Water Systems, page 44
[3] Rodgers, M. 2014. Impact of Corrosion Control on Publicly Owned Treatment Works. In Proceedings of the Water Quality and Technology Conference. AWWA. Denver, CO.
[4] Wessex Water, UK Study